Heat transfer in agitated vessels is a critical process in many industrial applications.
Convection plays a crucial role, particularly through forced convection induced by mixers or agitators.
This mechanism enhances the efficiency of heat transfer by maintaining a uniform temperature distribution and promoting the expulsion of gases.
As a result, it facilitates a quicker distillation process.
Mechanism of Heat Transfer in Agitated Vessels: 5 Key Factors
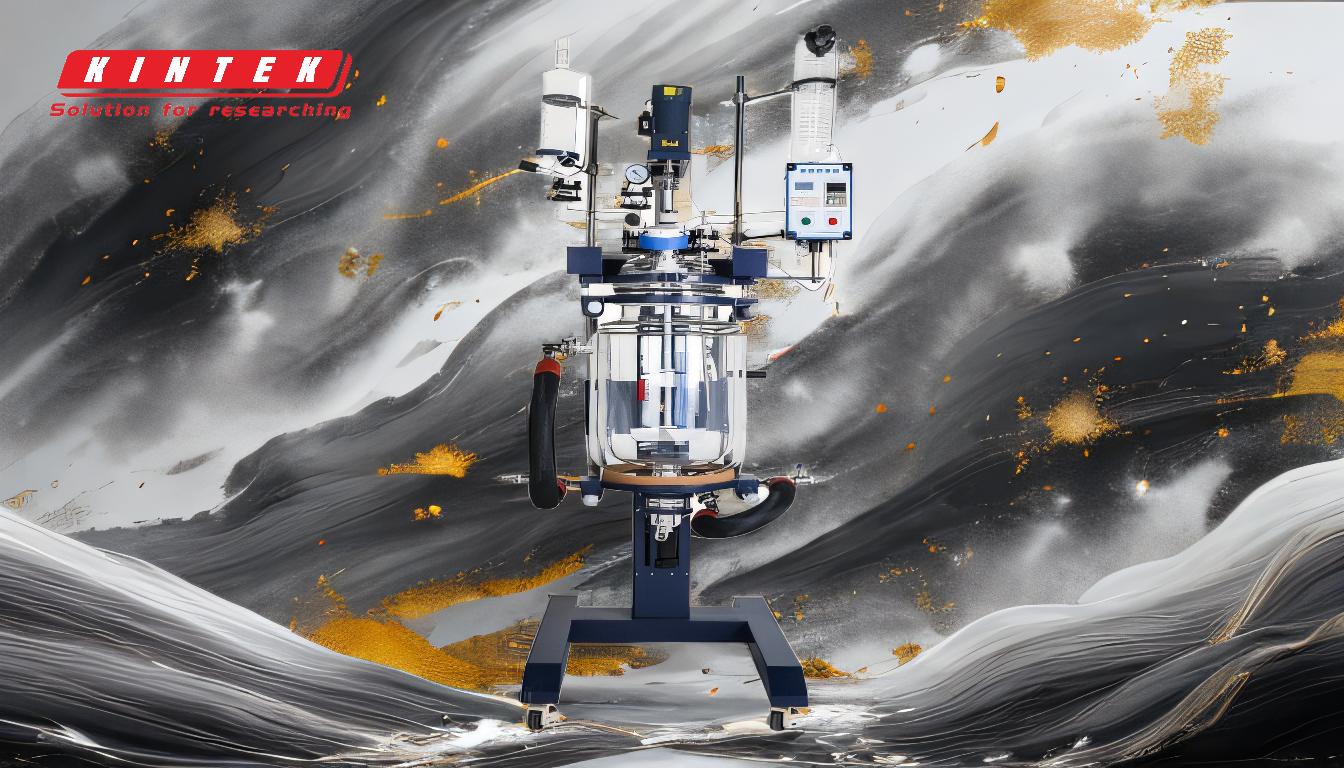
1. Forced Convection
In agitated vessels, the primary method of heat transfer is through forced convection.
This occurs when mechanical devices like mixers or agitators are used to keep the liquid in motion.
The movement of the liquid ensures that the heat, which is initially concentrated in the outer layers of the liquid near the heat source, is distributed throughout the liquid.
This continuous mixing prevents stratification of temperatures and ensures a more uniform heat distribution.
2. Use of Baffles
Most batch reactors incorporate baffles, which are stationary blades that disrupt the flow patterns created by the agitator.
These baffles help in breaking up large flow patterns, thereby enhancing the mixing efficiency and improving heat transfer.
They are typically fixed to the vessel cover or mounted on the interior of the side walls.
3. Impact of Agitator Design and Energy Input
The design of the agitator blades and the amount of energy applied significantly affect the efficiency of heat transfer.
In large vessels, excessive energy input (more than 5 W/L) can strain the cooling system and lead to stability issues with the agitator shaft.
Therefore, optimizing the agitator design and energy input is crucial for effective heat transfer without causing operational problems.
4. Cooling and Heating Systems
The internal temperature of the agitated vessel is managed through integrated cooling and heating systems.
These systems use pipes to either remove or add heat to the product inside the vessel, maintaining the desired temperature.
An external cooling system is often preferred due to its ease of cleaning and maintenance.
5. Advantages of Modern Agitated Vessels
Modern agitated vessels are designed with advanced features such as space-saving designs, leak-free construction, and wide operational temperature and pressure ranges.
These enhancements not only improve the efficiency of heat transfer but also make the vessels more robust and reliable.
In summary, the heat transfer in agitated vessels is predominantly managed through forced convection, aided by the strategic use of baffles and optimized agitator designs.
The integration of efficient cooling and heating systems ensures that the products inside the vessel are maintained at the desired temperature, enhancing the overall productivity and efficiency of the process.
Continue Exploring, Consult Our Experts
Unlock the Full Potential of Your Agitated Vessels with KINTEK Solutions!
Are you looking to enhance the heat transfer efficiency in your agitated vessels?
KINTEK offers cutting-edge solutions that optimize forced convection, baffle systems, and agitator designs to ensure uniform temperature distribution and efficient distillation processes.
Our advanced cooling and heating systems are designed for reliability and ease of maintenance, ensuring your operations run smoothly and productively.
Don't miss out on improving your process efficiencies.
Contact KINTEK today to learn more about our innovative products and how they can benefit your laboratory!