Methane pyrolysis is an effective method for converting methane, a potent greenhouse gas, into hydrogen and solid carbon.
This process helps reduce emissions and provides a cleaner source of hydrogen fuel.
Methane pyrolysis involves the thermal decomposition of methane at high temperatures.
Typically, this process is catalyzed by materials like nickel and operates at temperatures ranging from 500°C to over 1000°C.
Unlike steam methane reforming (SMR), which also produces hydrogen but results in carbon dioxide emissions, methane pyrolysis ideally produces no CO2 emissions.
This makes it a more environmentally friendly option.
1. Mechanism and Efficiency
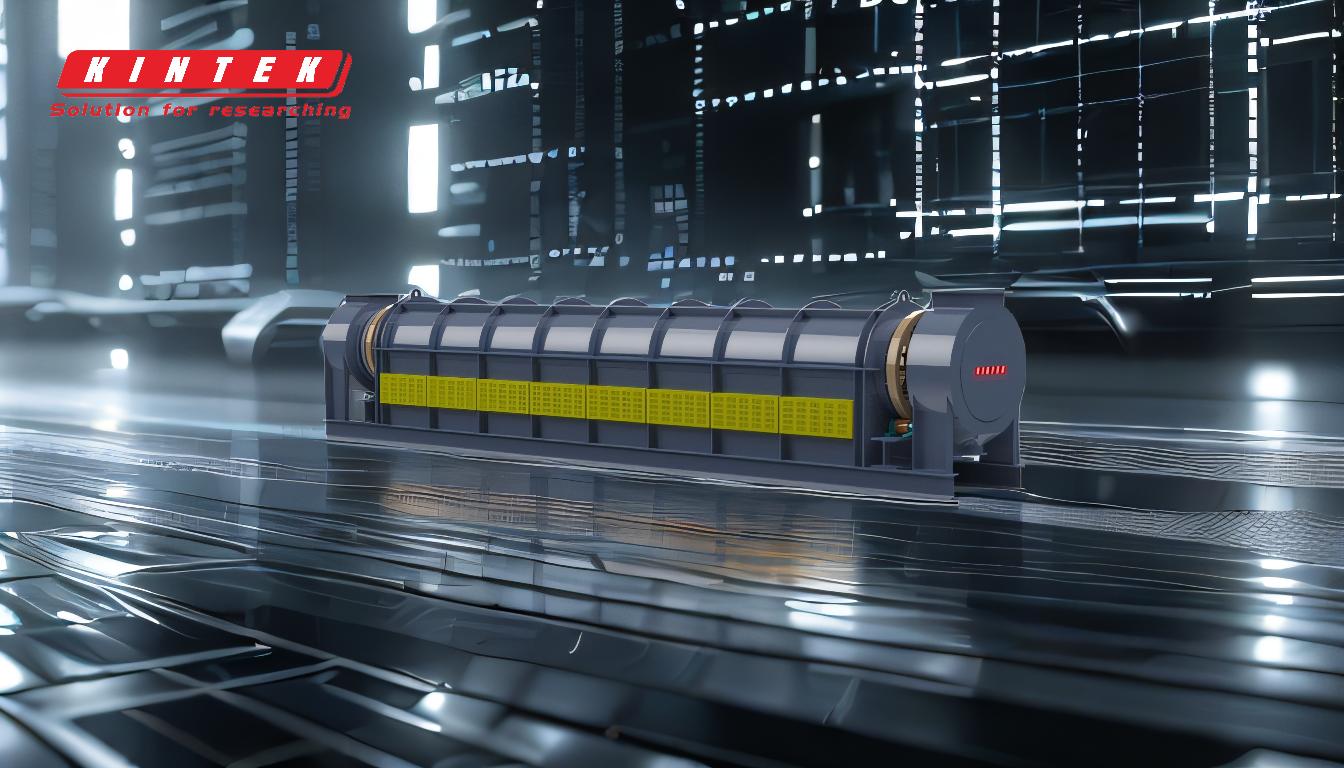
Methane pyrolysis involves breaking down methane (CH4) into its constituent elements: carbon and hydrogen.
The process is endothermic, requiring significant heat to initiate and sustain the reaction.
With catalysts like nickel, the reaction can occur at temperatures around 500°C.
For higher conversion rates, temperatures must be increased to over 800°C for catalytic processes and 1000°C for thermal processes.
The use of plasma torches can achieve temperatures up to 2000°C, enhancing the reaction rates.
The primary reaction of methane pyrolysis is: [ CH_4 \rightarrow C + 2H_2 ].
This reaction produces solid carbon and gaseous hydrogen, with the carbon being a potentially valuable byproduct rather than a pollutant.
2. Environmental and Economic Benefits
Methane pyrolysis offers several advantages over traditional methods like SMR.
By producing hydrogen without CO2 emissions, it significantly reduces the environmental impact associated with methane.
Methane accounts for about 20% of global greenhouse gas emissions.
The process also incentivizes the capture of methane from various sources, such as oil and gas sites, livestock farms, and landfills.
This turns a pollutant into a valuable resource.
The solid carbon produced can be used in various industries, reducing waste and providing an additional economic incentive for the adoption of methane pyrolysis technologies.
This dual output of hydrogen and carbon makes the process economically viable and environmentally beneficial.
3. Challenges and Future Prospects
Despite its advantages, methane pyrolysis faces challenges such as the need for high temperatures.
These high temperatures can increase energy costs and technical complexity.
Additionally, the process must be designed to handle a natural gas stream that includes other gases.
This ensures that these are also effectively managed to prevent the emission of hazardous gases.
In the coming years, advancements in catalyst technology and process optimization are expected to make methane pyrolysis more efficient and cost-effective.
This could potentially lead to its widespread adoption as a key method for hydrogen production and greenhouse gas reduction.
Continue exploring, consult our experts
Discover the future of sustainable energy with KINTEK SOLUTION’s cutting-edge methane pyrolysis technology.
By converting methane into clean hydrogen and solid carbon, we are not just reducing greenhouse gas emissions but also paving the way for a greener planet.
Explore our high-performance materials and innovative solutions today, and be a part of the revolution towards a cleaner, more efficient energy landscape.
Join us in shaping the future of hydrogen production and emission reduction with KINTEK SOLUTION – your partner in green innovation!