Increasing the pressure in a chemical reaction can have several effects on the reaction rate.
Firstly, increasing the pressure will increase the number of reactant particles per unit volume.
This means that the collision rate between the particles will also increase.
Since reactions occur when reactant particles collide with sufficient energy, the increased collision rate will lead to more successful collisions and therefore a higher reaction rate.
Secondly, higher pressures compress reactant molecules, resulting in a considerable rise in their concentration inside the reaction vessel.
This increased concentration makes molecular collisions more likely to occur, further speeding up the reaction.
Additionally, elevated pressures can alter the activation energy necessary for a reaction to occur.
This can result in a shift in the reaction pathway, potentially opening up new pathways that have lower activation energies.
Lowering the energy barrier enables the reaction to proceed more quickly, leading to an increased reaction rate.
It is important to note that increasing pressure can also impact the decomposition of reagents and starting materials, especially if it involves the release or reaction with a gas.
However, when the desired reaction is accelerated, competing reactions are minimized, and pressure generally enables faster reactions with cleaner reaction profiles.
Pressure reactors, such as high-pressure glass reactors, play a crucial role in various chemical applications.
They allow reactions to be carried out at higher temperatures than at atmospheric pressure, which can further accelerate the reaction rate.
Additionally, pressure reactors can push chemical equilibrium towards the side of the reaction with fewer moles of gas, potentially increasing yield.
These reactors are used in hydrogenations, gas phase reactions, catalysis, corrosion testing, supercriticality studies, and hydrothermal syntheses.
In summary, increasing pressure in a chemical reaction increases the concentration and collision frequency of reactant particles, alters the activation energy, and can shift the reaction pathway, all of which contribute to an increase in the reaction rate.
Elevate your reaction rates with KINTEK's pressure reactors!
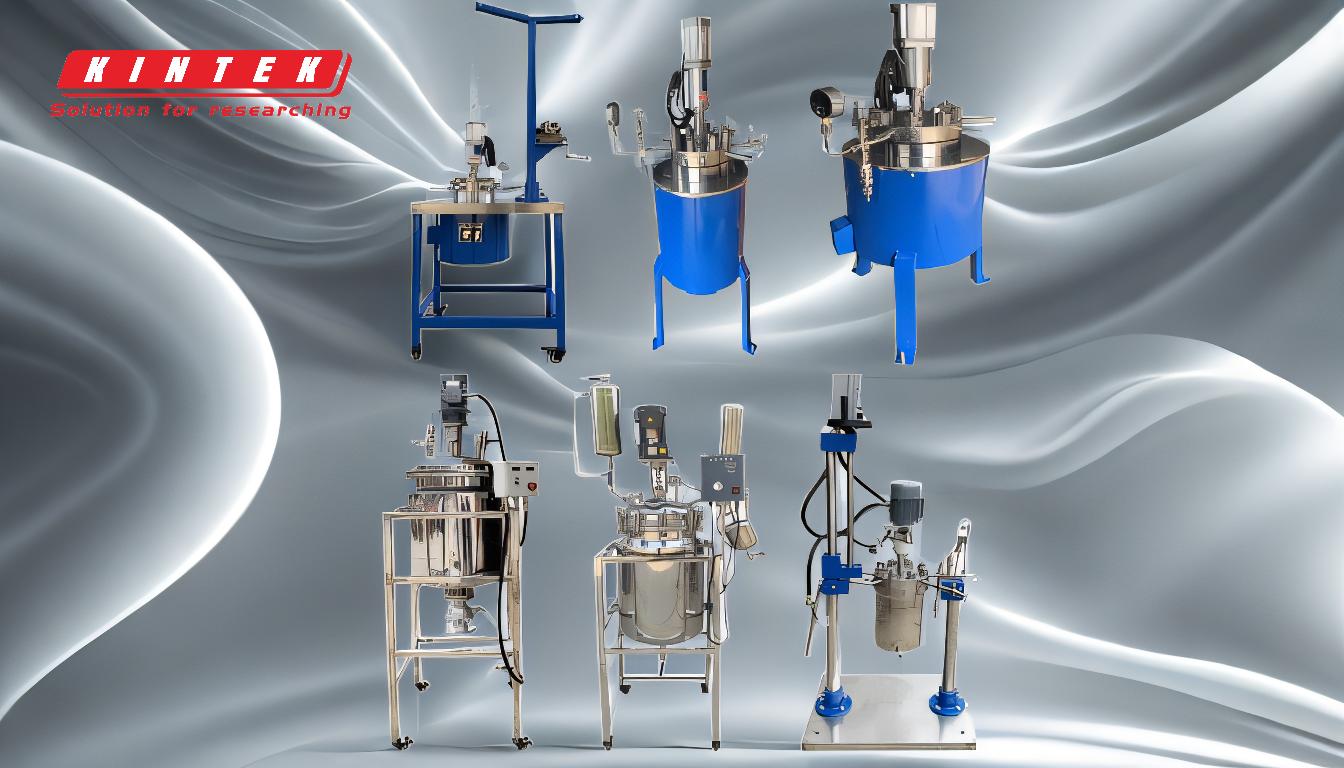
Our high-quality equipment increases the collision rate and concentration of reactant molecules, leading to faster reactions and improved yields.
Discover new reaction pathways and enhance your chemical applications with KINTEK.
Contact us today and unlock the power of pressure!
Continue exploring, consult our experts
Interested in learning more about how pressure can enhance your chemical reactions?
Contact our experts today to discuss your specific needs and discover how KINTEK's pressure reactors can benefit your research and production processes.
Don't miss out on the opportunity to elevate your reaction rates and achieve better results.
Get in touch now!