X-ray Fluorescence (XRF) technology is a powerful analytical tool known for its low interference, high-speed analysis, multi-element detection, and non-destructive testing capabilities. The limits of detection (LOD) for XRF vary depending on factors such as the element being analyzed, the instrument's sensitivity, and the sample matrix. Generally, XRF can detect elements at concentrations ranging from parts per million (ppm) to low percentage levels. The technology's ability to provide fast, accurate, and non-destructive multi-element analysis makes it invaluable in fields like materials science, environmental science, and life sciences. However, the LOD is influenced by the instrument's design, the energy of the X-ray source, and the sample preparation method.
Key Points Explained:
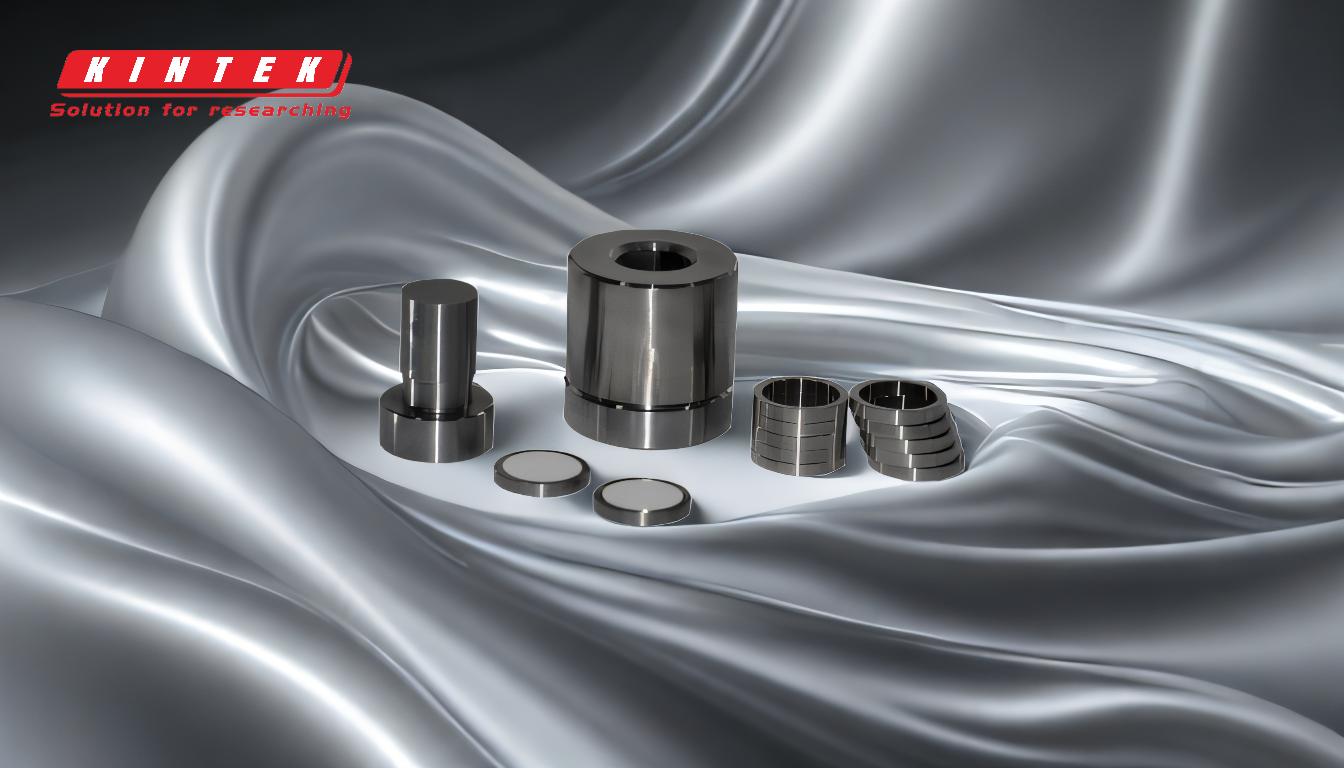
-
Low Interference and High Sensitivity:
- XRF technology benefits from low interference due to the unique X-ray fluorescence signals emitted by each element. This ensures high accuracy in detection.
- The absence of a continuous spectrum and small background intensity in XRF spectra result in a high peak-to-base ratio, enhancing sensitivity and improving the limits of detection.
- For most elements, XRF can achieve detection limits in the ppm range, making it suitable for trace element analysis.
-
High-Speed Analysis and Multi-Element Detection:
- XRF allows for rapid analysis of large sample volumes, which is crucial for high-throughput environments.
- The ability to detect multiple elements simultaneously in complex material systems makes XRF a versatile tool for various applications.
- The detection range typically spans from light elements (e.g., sodium) to heavy elements (e.g., uranium), with detection limits varying based on the element's atomic number and the instrument's configuration.
-
Non-Destructive Testing:
- One of the most significant advantages of XRF is its non-destructive nature, which preserves sample integrity and reduces preparation complexity.
- This feature is particularly beneficial in fields like archaeology, art conservation, and quality control, where preserving the sample is critical.
- The non-destructive aspect also allows for repeated measurements, improving the reliability of the results.
-
Sample Preparation and Matrix Effects:
- XRF requires minimal sample preparation, making it suitable for various solid and liquid substances.
- However, matrix effects, such as absorption and inter-element excitation, can influence the detection limits. These effects are relatively easier to correct in XRF compared to other techniques, especially for heavier elements.
- For light elements, chemical bonds and matrix effects can slightly reduce sensitivity, but advancements in instrumentation and software have mitigated these challenges.
-
Instrumentation and Technological Advancements:
- The sensitivity and detection limits of XRF are heavily dependent on the instrument's design, including the X-ray source energy and detector type.
- Modern XRF instruments, equipped with advanced detectors and high-energy X-ray tubes, can achieve lower detection limits and higher precision.
- The integration of artificial intelligence (AI), machine learning (ML), and cloud computing has further enhanced XRF analysis by improving calibration, data processing, and result interpretation.
-
Applications and Practical Limits:
- XRF is widely used in materials science, environmental monitoring, and life sciences due to its fast, accurate, and economical multi-element analysis capabilities.
- In practical applications, the detection limits for XRF typically range from 1 ppm to 0.1%, depending on the element and sample matrix.
- For example, in the analysis of FeSi alloys, XRF provides high throughput and reliable results, making it an ideal alternative to more complex techniques.
In summary, the limits of detection for XRF are influenced by the element being analyzed, the instrument's sensitivity, and the sample matrix. While XRF excels in providing fast, accurate, and non-destructive multi-element analysis, achieving the lowest detection limits often requires careful consideration of these factors. Advances in instrumentation and data analysis techniques continue to push the boundaries of what XRF can achieve, making it an indispensable tool in modern analytical science.
Summary Table:
Factor | Impact on Detection Limits |
---|---|
Element Analyzed | Detection limits vary by atomic number; lighter elements may have slightly higher LODs. |
Instrument Sensitivity | Advanced detectors and high-energy X-ray sources improve precision and lower LODs. |
Sample Matrix | Matrix effects like absorption can influence LODs, but are easier to correct in XRF. |
Sample Preparation | Minimal preparation required, preserving sample integrity and enabling repeated measurements. |
Technological Advancements | AI, ML, and cloud computing enhance calibration, data processing, and result interpretation. |
Practical Applications | LODs typically range from 1 ppm to 0.1%, making XRF ideal for trace element analysis. |
Discover how XRF can revolutionize your analytical processes—contact our experts today!