Carbon nanotubes (CNTs) are indeed electrical conductors. This property is a fundamental aspect of their nanoscale properties, which include excellent mechanical, thermal, and electrical conductivity.
4 Key Points to Understand
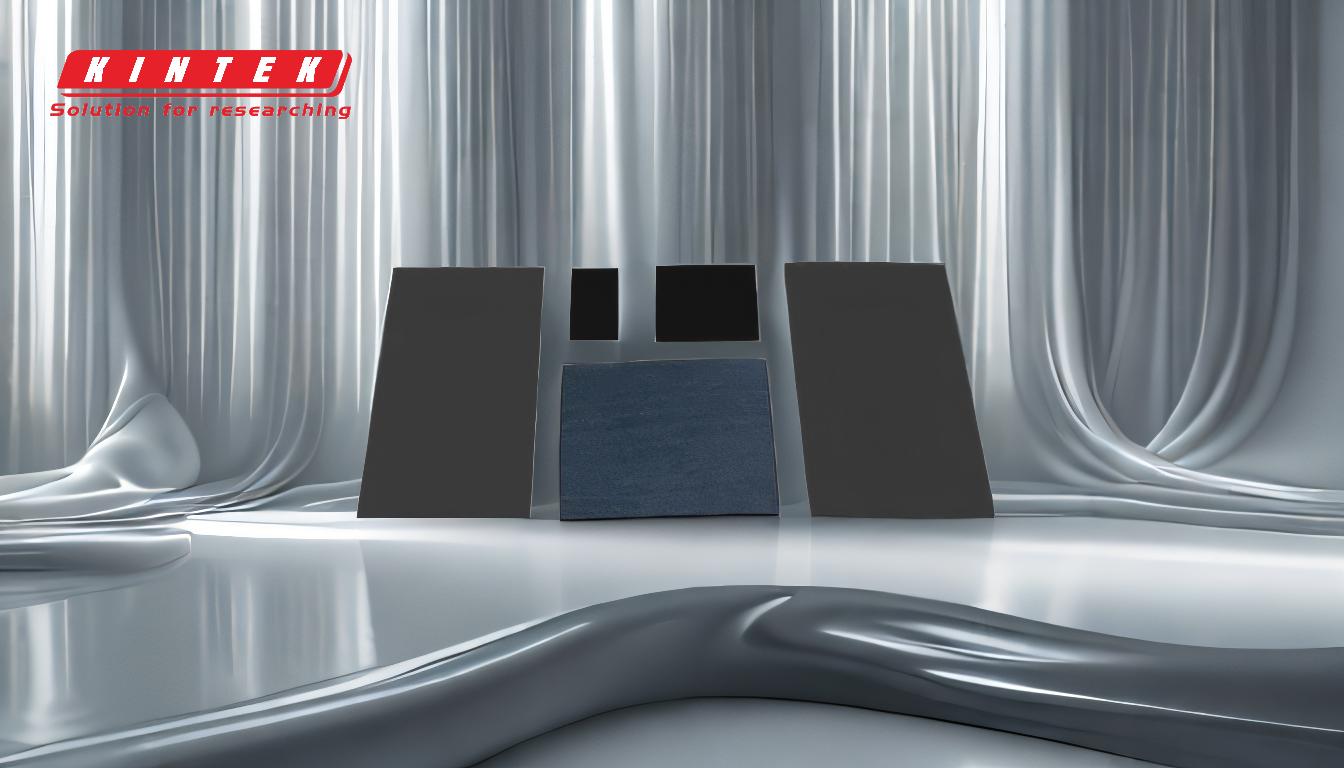
1. Electrical Conductivity of CNTs
Carbon nanotubes exhibit high electrical conductivity due to their unique structure. They are composed of carbon atoms arranged in a hexagonal lattice rolled into a seamless tube. This structure allows electrons to move freely along the length of the nanotube, making them excellent conductors of electricity. The conductivity of CNTs is comparable to that of metals and is superior to many other carbon-based materials.
2. Applications in Energy Storage
The reference highlights the use of CNTs as conductive additives in lithium-ion batteries and ultracapacitors. By incorporating CNTs into the electrodes, the conductivity of these components is significantly enhanced. This improvement not only boosts the energy density but also improves the mechanical properties of the electrodes, allowing for thicker electrodes and broader operational temperature ranges. The enhanced conductivity aids in faster electron transfer during charging and discharging, which is critical for the efficiency and lifespan of these energy storage devices.
3. Comparison with Other Carbon-Based Materials
When considering the environmental impact and performance, CNTs are often compared to other carbon-based materials like carbon black and graphene. Carbon black, used extensively in various applications including tires, typically has higher CO2 emissions and requires higher loading in composites compared to CNTs. Graphene, another highly conductive material, faces challenges in its production methods, including energy efficiency and the use of harsh chemicals. CNTs, therefore, offer a more sustainable and efficient alternative in many applications due to their superior conductivity and lower environmental impact.
4. Technological Advancements
The report also discusses the technological advancements in the production and post-processing of CNTs. These advancements are crucial for realizing the full potential of CNTs in various applications. The ability to effectively functionalize, purify, and separate CNTs, along with improving their dispersion, is essential for enhancing their performance and integration into different systems.
Continue exploring, consult our experts
Discover the cutting-edge advantages of carbon nanotubes with KINTEK SOLUTION! Harness their unparalleled electrical conductivity and explore their pivotal role in revolutionizing energy storage technologies. From efficient battery and capacitor enhancements to sustainable alternatives in the carbon-based materials market, elevate your research and development with our premium CNTs. Join us in driving technological advancements and unlocking the full potential of these remarkable nanoscale wonders. Trust KINTEK SOLUTION for your next breakthrough!