Chemical vapor deposition (CVD) is a widely used method for synthesizing high-quality graphene.
It is particularly suitable for large-scale production.
This method involves the decomposition of hydrocarbon precursors on a transition metal substrate.
This leads to the formation of graphene layers.
The choice of substrate, such as copper, nickel, or cobalt, significantly influences the quality and uniformity of the graphene produced.
4 Key Factors to Consider
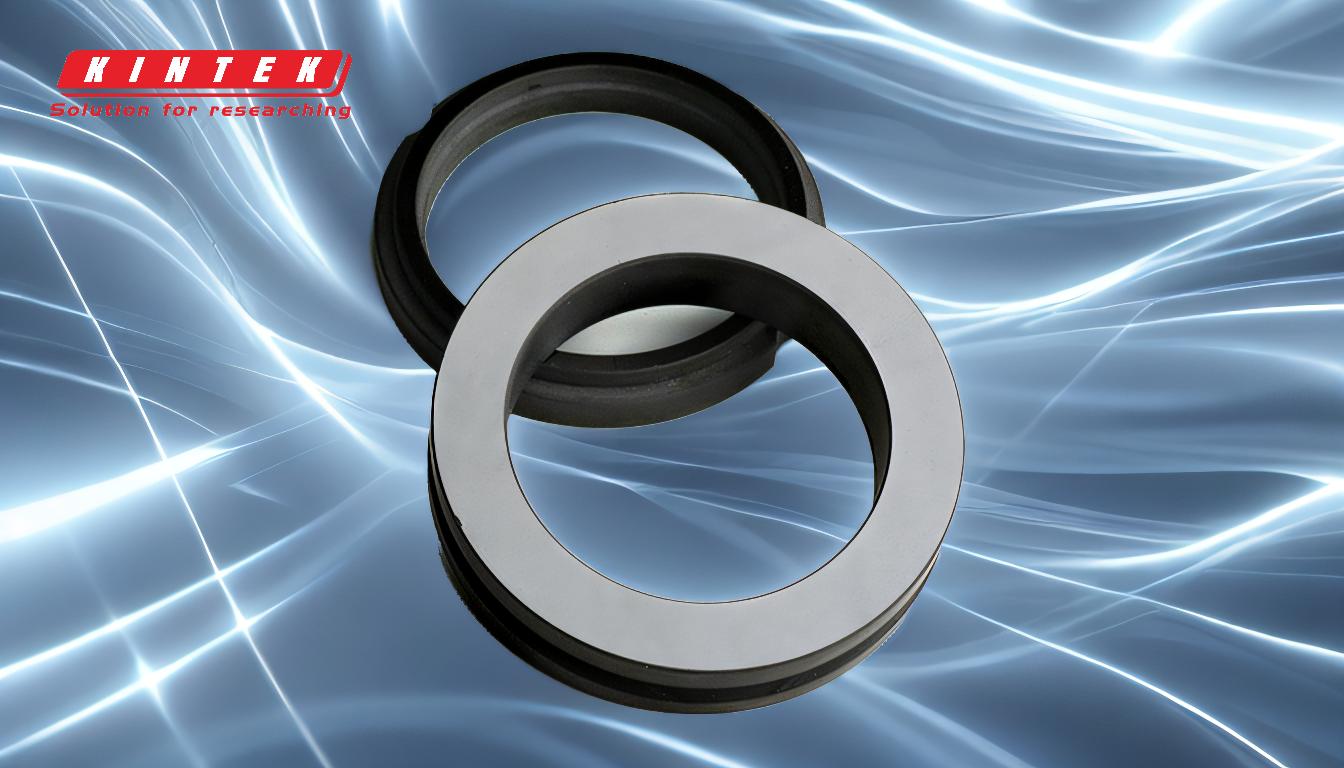
1. Substrate Selection
The choice of substrate in CVD is crucial.
It affects the graphene's properties and the ease of its transfer.
Copper is often preferred due to its ability to support the exclusive deposition of graphene monolayers.
Nickel, on the other hand, allows for controlled formation of graphene layers but may lead to multilayer growth.
Cobalt and other transition metals like ruthenium, iridium, platinum, rhodium, gold, palladium, and rhenium have also been explored.
However, they generally do not match the efficiency of copper, nickel, and cobalt in terms of cost, quality, and scalability.
2. Process Parameters
The CVD process requires careful control of parameters such as gas volumes, pressure, temperature, and time duration.
This ensures high-quality graphene production.
The hydrocarbon precursors decompose at high temperatures, releasing carbon radicals that then form graphene layers on the substrate surface.
The metal substrate acts as a catalyst, lowering the reaction's energy barrier and influencing the deposition mechanism.
3. Applications and Advantages
CVD-produced graphene is highly valued for applications in high-performance electronics and sensors.
This is due to its low defect count and good uniformity.
The method's ability to produce large-area graphene makes it particularly suitable for industrial applications where scalability is essential.
4. Comparison with Other Methods
While other methods like mechanical exfoliation, liquid-phase exfoliation, and reduction of graphene oxide exist, CVD stands out for its potential to produce high-quality, large-scale graphene.
These other methods may offer advantages in specific contexts, such as simplicity or lower equipment requirements.
However, they often lack the scalability and uniformity of CVD.
Continue exploring, consult our experts
Unlock the Full Potential of Graphene Production with KINTEK SOLUTION!
Our advanced CVD systems are designed to refine every aspect of graphene synthesis.
From the selection of optimal substrates to the fine-tuning of critical process parameters.
Embrace the future of high-performance electronics and sensors by joining our cutting-edge laboratory supply solutions.
Elevate your graphene production with KINTEK SOLUTION today!