Carbon nanotubes (CNTs) are often celebrated for their exceptional electrical conductivity, but there are scenarios where they may not conduct electricity as expected. This phenomenon can be attributed to factors such as structural defects, impurities, or the specific type of nanotube (metallic vs. semiconducting). Understanding these factors is crucial for applications in electronics, materials science, and nanotechnology. Below, we explore the reasons why carbon nanotubes might not conduct electricity, breaking down the key points for clarity.
Key Points Explained:
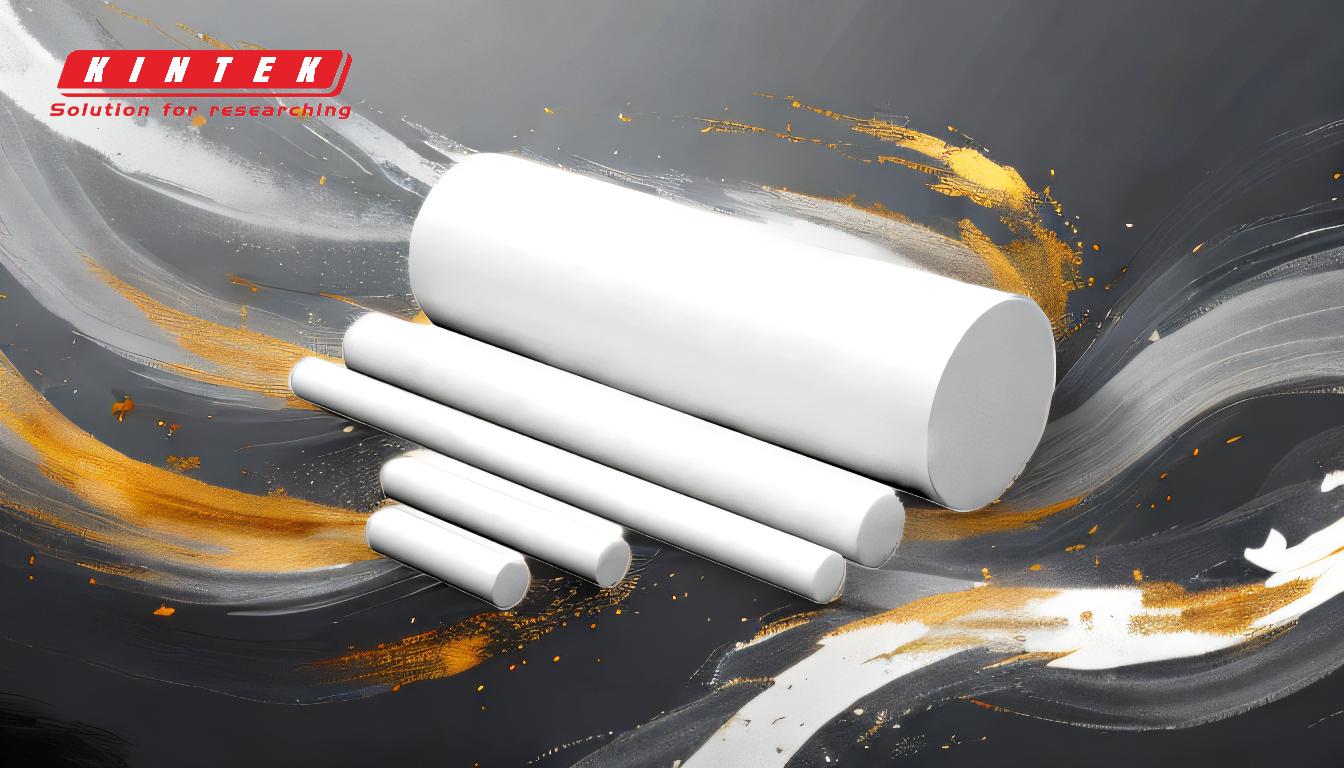
-
Structural Defects in Carbon Nanotubes
- Carbon nanotubes derive their electrical properties from their perfect hexagonal lattice structure. However, defects such as vacancies, Stone-Wales defects (a rearrangement of carbon bonds), or kinks in the nanotube can disrupt this lattice.
- These defects act as scattering centers for electrons, impeding their flow and reducing conductivity. In severe cases, defects can completely block electrical conduction.
-
Impurities and Contaminants
- During the synthesis of carbon nanotubes, impurities like metal catalysts or amorphous carbon can become embedded in the nanotube structure.
- These impurities can introduce energy barriers or trap electrons, hindering their movement and reducing the overall conductivity of the nanotube.
-
Type of Carbon Nanotube: Metallic vs. Semiconducting
- Carbon nanotubes can be either metallic or semiconducting, depending on their chirality (the way the graphene sheet is rolled). Metallic nanotubes conduct electricity efficiently, while semiconducting nanotubes have a bandgap that limits conductivity.
- If a nanotube is semiconducting, it will not conduct electricity under certain conditions, such as at low temperatures or without an applied voltage to overcome the bandgap.
-
Environmental Factors
- Exposure to oxygen, moisture, or other reactive gases can chemically alter the surface of carbon nanotubes, forming insulating layers or functional groups that reduce conductivity.
- Mechanical stress or bending can also deform the nanotube structure, leading to reduced electrical performance.
-
Contact Resistance at Interfaces
- When carbon nanotubes are integrated into devices, the interfaces between the nanotube and electrodes or other materials can introduce contact resistance.
- Poor contact quality, misalignment, or incompatible materials can significantly reduce the effective conductivity of the nanotube in a practical application.
-
Diameter and Length Effects
- The diameter and length of a carbon nanotube can influence its electrical properties. Thinner nanotubes may exhibit quantum confinement effects, altering their conductivity.
- Longer nanotubes are more prone to defects and impurities, which can degrade their electrical performance over extended lengths.
By addressing these factors, researchers and engineers can optimize the design, synthesis, and integration of carbon nanotubes to achieve the desired electrical properties for specific applications.
Summary Table:
Factor | Impact on Conductivity |
---|---|
Structural Defects | Disrupts lattice, acts as electron scattering centers, reduces or blocks conductivity. |
Impurities and Contaminants | Introduces energy barriers, traps electrons, reduces overall conductivity. |
Metallic vs. Semiconducting | Semiconducting nanotubes have a bandgap, limiting conductivity under certain conditions. |
Environmental Factors | Chemical alterations or mechanical stress reduce conductivity. |
Contact Resistance at Interfaces | Poor contact quality or misalignment increases resistance, reducing effective conductivity. |
Diameter and Length Effects | Thinner or longer nanotubes may exhibit reduced conductivity due to quantum effects or defects. |
Need help optimizing carbon nanotubes for your project? Contact our experts today for tailored solutions!