X-ray Fluorescence (XRF) is a widely used analytical technique for determining the elemental composition of materials. While it offers numerous advantages, such as non-destructive testing, rapid analysis, and the ability to analyze a wide range of elements, it also has several limitations and drawbacks. These include issues related to sensitivity, matrix effects, sample preparation, and the inability to detect light elements effectively. Understanding these drawbacks is crucial for users to make informed decisions about when and how to use XRF in their analytical workflows.
Key Points Explained:
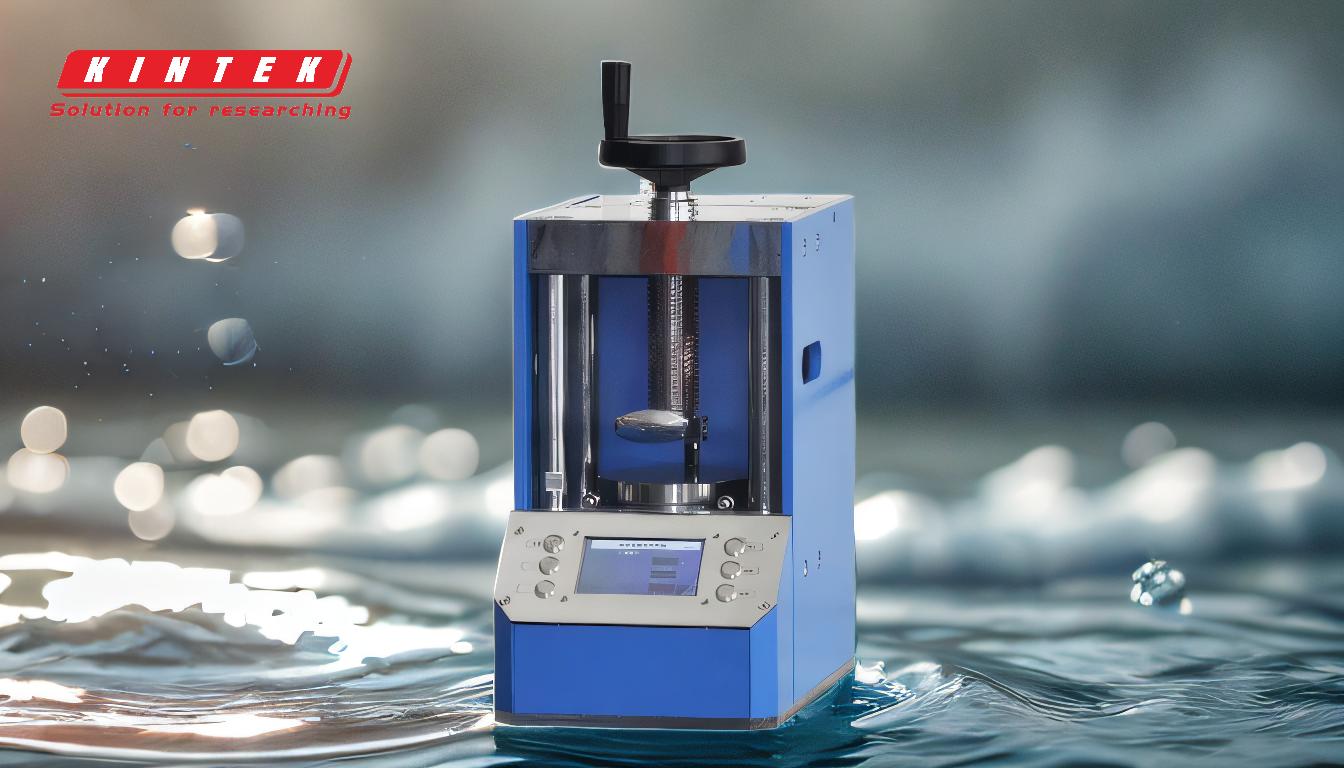
-
Limited Sensitivity for Light Elements:
- XRF is less effective at detecting light elements (those with atomic numbers below 11, such as hydrogen, helium, and lithium). This is because the fluorescence yield for these elements is very low, making it difficult to obtain accurate measurements. For example, detecting carbon or oxygen in a sample can be challenging, which limits the technique's applicability in certain fields like organic chemistry or environmental science where light elements are prevalent.
-
Matrix Effects:
- The accuracy of XRF measurements can be significantly affected by the matrix of the sample. Matrix effects occur when the composition of the sample influences the intensity of the emitted X-rays. For instance, a high concentration of one element can interfere with the detection of another element, leading to inaccurate results. This makes it necessary to use matrix-matched standards or perform complex corrections, which can be time-consuming and require specialized knowledge.
-
Sample Preparation Requirements:
- While XRF is often considered a non-destructive technique, certain types of samples may require extensive preparation to obtain accurate results. For example, solid samples may need to be ground into a fine powder and pressed into pellets, or liquids may need to be filtered and homogenized. This preparation can introduce errors or contamination, and in some cases, may alter the sample's original state, which is a drawback for those seeking truly non-destructive analysis.
-
Detection Limits and Sensitivity:
- XRF has higher detection limits compared to some other analytical techniques like Inductively Coupled Plasma Mass Spectrometry (ICP-MS). This means that XRF may not be suitable for detecting trace elements at very low concentrations. For example, in environmental monitoring, where detecting parts-per-billion (ppb) levels of contaminants is often required, XRF may not provide the necessary sensitivity.
-
Interference from Overlapping Peaks:
- In XRF spectra, peaks from different elements can overlap, making it difficult to distinguish between them. This is particularly problematic when analyzing complex samples with multiple elements. For instance, the Kα line of one element might overlap with the Lα line of another, leading to misinterpretation of the data. Advanced software and deconvolution techniques are often required to resolve these overlaps, which adds to the complexity of the analysis.
-
Inability to Provide Chemical State Information:
- XRF provides information about the elemental composition of a sample but does not offer details about the chemical state or molecular structure of the elements. For example, it cannot distinguish between different oxidation states of an element, which can be crucial in fields like materials science or catalysis. This limitation means that XRF must often be used in conjunction with other techniques, such as X-ray Photoelectron Spectroscopy (XPS), to obtain a complete picture of the sample.
-
Cost and Complexity of Equipment:
- While portable XRF devices are available and relatively easy to use, high-performance benchtop XRF instruments can be expensive and require significant expertise to operate and maintain. The need for regular calibration, maintenance, and the use of consumables like X-ray tubes or detectors can add to the overall cost of ownership. Additionally, the interpretation of XRF data often requires specialized software and trained personnel, which can be a barrier for smaller laboratories or those with limited resources.
-
Radiation Safety Concerns:
- XRF instruments generate X-rays, which pose potential health risks if not handled properly. Users must adhere to strict safety protocols, including the use of shielding and personal protective equipment, to minimize exposure. This adds an additional layer of complexity and responsibility for laboratories using XRF, particularly in environments where multiple users are involved or where portable XRF devices are used in the field.
In summary, while XRF is a powerful and versatile analytical tool, it is not without its limitations. Users must carefully consider these drawbacks, particularly when dealing with light elements, complex matrices, or trace-level analyses. By understanding these limitations, analysts can better determine when XRF is the appropriate technique for their specific application and when alternative methods might be more suitable.
Summary Table:
Drawback | Description |
---|---|
Limited Sensitivity for Light Elements | XRF struggles to detect elements with atomic numbers below 11 (e.g., hydrogen, carbon). |
Matrix Effects | Sample composition can interfere with XRF measurements, requiring complex corrections. |
Sample Preparation Requirements | Some samples need extensive preparation, potentially altering their original state. |
Detection Limits and Sensitivity | XRF may not detect trace elements at very low concentrations (e.g., ppb levels). |
Overlapping Peaks | Elemental peaks can overlap, complicating data interpretation. |
No Chemical State Information | XRF cannot distinguish between oxidation states or molecular structures. |
Cost and Complexity of Equipment | High-performance XRF instruments are expensive and require expertise to operate. |
Radiation Safety Concerns | XRF generates X-rays, necessitating strict safety protocols. |
Need help deciding if XRF is right for your application? Contact our experts today!