Heat transfer in a vacuum primarily occurs through radiation.
Unlike conduction and convection, which require a medium to transfer heat, radiation involves the transfer of heat energy through electromagnetic waves.
These waves can travel through a vacuum without the need for any intervening matter.
5 Key Insights
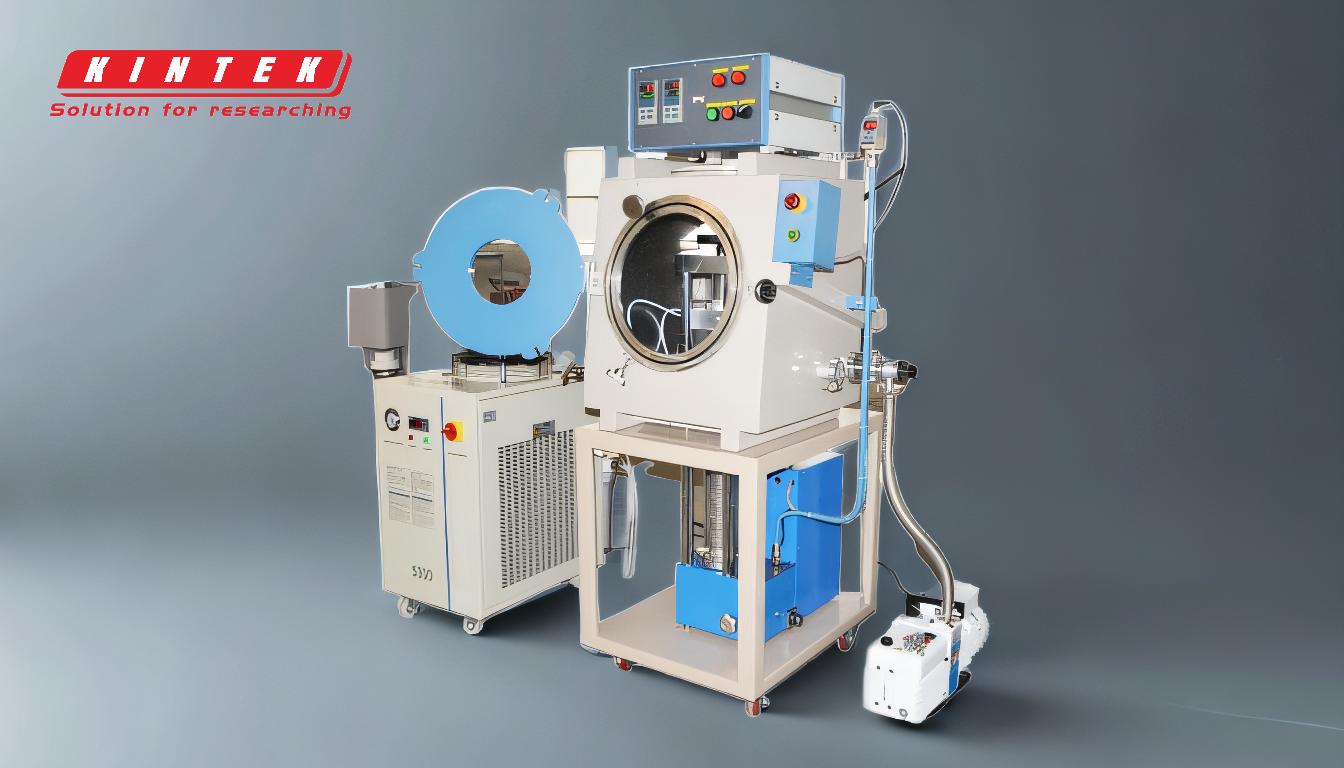
1. Radiation as the Primary Mode of Heat Transfer in a Vacuum
Radiation is the emission of energy as electromagnetic waves or particles.
In the context of heat transfer, these waves are typically in the form of infrared radiation, although they can also include visible light and other forms of electromagnetic radiation.
The key characteristic of radiation is that it does not require a medium to propagate; it can travel through empty space, making it the only effective method of heat transfer in a vacuum.
2. Mechanism of Radiation
When an object is heated, it emits radiation in the form of electromagnetic waves.
The intensity of this radiation is governed by the Stefan-Boltzmann law, which states that the power emitted per unit area of a black body (an idealized physical body that absorbs all incident electromagnetic radiation) is proportional to the fourth power of the absolute temperature of the body (e = C(T/100)^4, where e is the heat transfer capacity, T is the absolute temperature, and C is a constant).
This means that as the temperature of an object increases, the rate of heat transfer through radiation increases dramatically.
3. Examples in Space
In space, which is largely a vacuum, the primary method of heat transfer from the sun to the Earth is through radiation.
The sun emits electromagnetic waves, including visible light and infrared radiation, which travel through the vacuum of space and reach the Earth.
This radiation is then absorbed by the Earth, causing it to heat up.
4. Applications in Vacuum Heating
In industrial processes such as vacuum heat treatment, the vacuum environment is used to prevent oxidation, decarburization, and other undesirable chemical reactions that can occur in the presence of air.
In these processes, heat is transferred to the workpiece solely through radiation, which allows for precise control of the heating environment and can lead to improved material properties.
5. Summary
In summary, heat transfer in a vacuum occurs through radiation, a process that involves the emission and propagation of electromagnetic waves through space.
This mode of heat transfer is crucial in environments where other methods, such as conduction and convection, are not feasible due to the absence of a medium.
Continue exploring, consult our experts
Discover the power of advanced heat transfer technology with KINTEK SOLUTION.
Embrace the unparalleled efficiency of radiation heating in vacuum environments where traditional methods fail.
Our innovative products leverage the principles of electromagnetic wave propagation to provide precision and control in a range of industrial applications.
Trust KINTEK SOLUTION to unlock the full potential of your vacuum heat treatments and elevate your industrial processes to new heights.
Let us be your partner in revolutionizing heat transfer solutions.