The conductivity of materials is influenced by several factors.
Understanding these factors can help in selecting the right materials for specific applications.
What affects the conductivity of materials? 5 Key Factors to Consider
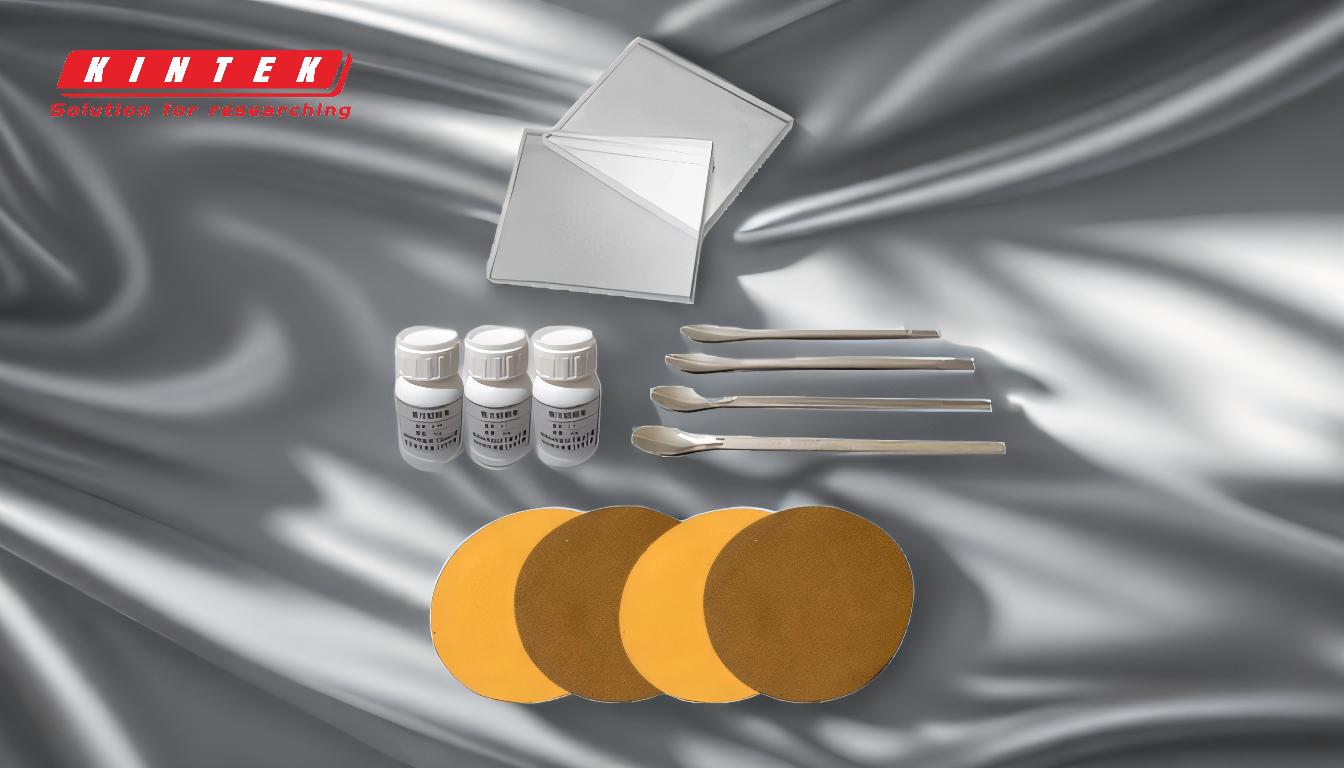
1. Ion Concentrations and Types
The concentrations of ions play a significant role in material conductivity.
Different types of ions present in a solution can also affect conductivity.
2. Temperature
Temperature is another crucial factor that influences material conductivity.
In the case of electrical properties, temperature can significantly alter the conductivity of a thin film.
3. Material Properties
The material of the film (metal, semiconductor, or insulator) and the substrate affect conductivity.
The size effect is important, where charge carriers in a thin film have a shorter mean free path compared to bulk materials.
This results in reduced electrical conductivity due to more scattering points like structural defects and grain boundaries.
4. Magnetic Properties
Magnetic materials generate heat through eddy currents and the hysteresis effect.
These materials lose their magnetic properties at a specific temperature known as the Curie point.
The resistance of magnetic materials is measured in terms of permeability, with non-magnetic materials having a permeability of 1 and magnetic materials having a permeability as high as 500.
5. Band Structure
The band structure of a material is a significant factor in conductivity.
Conductors have a very low energy difference between partially filled energy levels and empty levels, allowing for easy electron mobility.
Insulators have a forbidden band gap between the valence band and the conduction band, preventing the transmission of electrons.
Semiconductors have a smaller band gap compared to insulators, and their conductivity is directly related to temperature.
Continue exploring, consult our experts
Enhance your laboratory experiments with KINTEK's advanced conductivity measurement equipment!
Whether you're studying the impact of ions, temperature, magnetic properties, or material thickness on conductivity, our state-of-the-art instruments provide accurate and reliable results.
Don't miss out on the opportunity to discover new insights and unlock the potential of your research.
Upgrade your lab with KINTEK today!