Carbon nanotubes (CNTs) grow through mechanisms that involve the decomposition of carbon-containing gases on catalytic nanoparticles, followed by the diffusion of carbon atoms and their assembly into tubular structures. The growth process is influenced by factors such as temperature, catalyst type, and gas composition. The primary mechanisms include tip-growth and base-growth models, where the catalyst either remains at the tip of the growing nanotube or stays anchored to the substrate. Understanding these mechanisms is crucial for controlling the structure, quality, and properties of CNTs for various applications.
Key Points Explained:
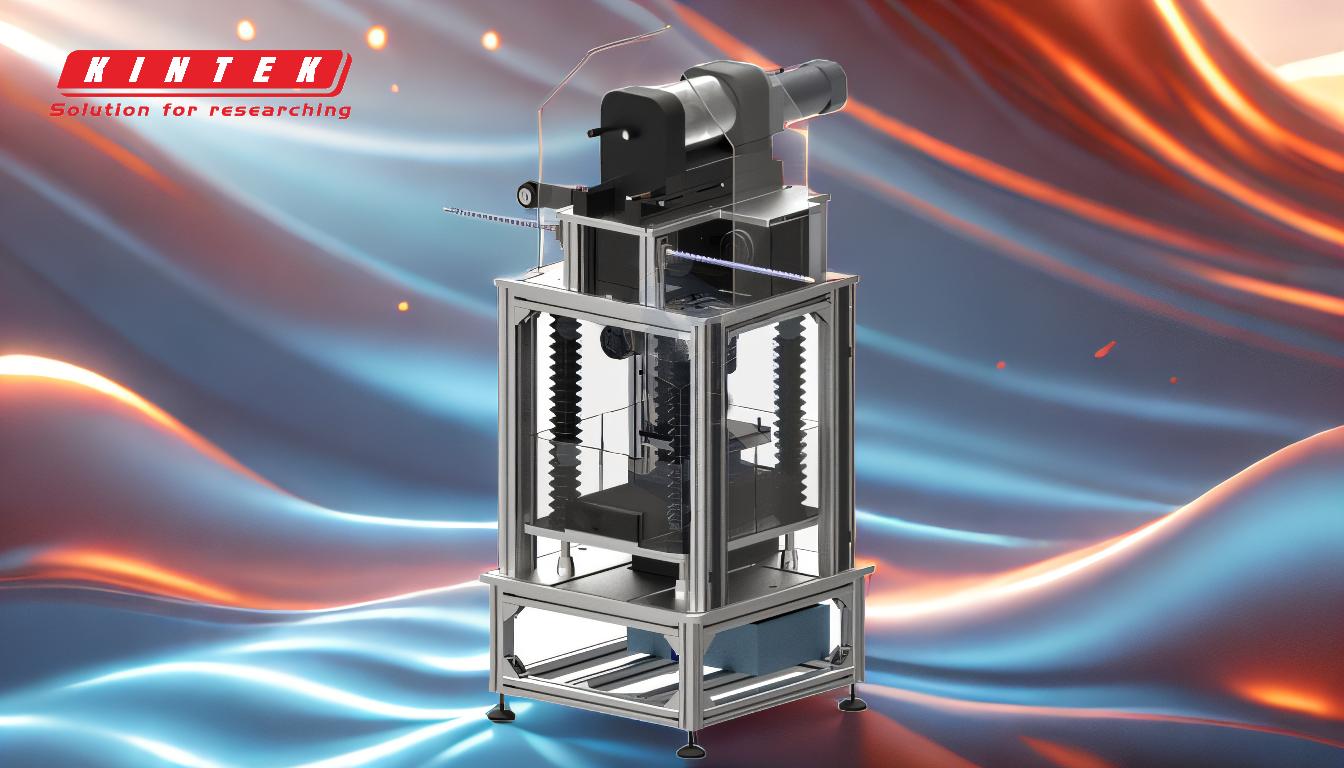
-
Catalytic Decomposition of Carbon Sources:
- Carbon nanotubes are typically synthesized using chemical vapor deposition (CVD), where a carbon-containing gas (e.g., methane, ethylene, or acetylene) decomposes on the surface of a metal catalyst (e.g., iron, nickel, or cobalt).
- The catalyst nanoparticles act as nucleation sites, breaking the carbon-carbon bonds in the gas molecules and releasing carbon atoms.
-
Carbon Diffusion and Assembly:
- The carbon atoms diffuse through or on the surface of the catalyst nanoparticles.
- These atoms then assemble into hexagonal carbon rings, forming the graphene-like structure of the nanotube walls.
-
Growth Models:
- Tip-Growth Mechanism: In this model, the catalyst particle is lifted from the substrate as the nanotube grows, remaining at the tip of the tube. This occurs when the adhesion between the catalyst and the substrate is weak.
- Base-Growth Mechanism: Here, the catalyst particle remains anchored to the substrate, and the nanotube grows upward from the particle. This happens when the adhesion between the catalyst and the substrate is strong.
-
Role of Temperature and Gas Composition:
- The growth temperature significantly affects the quality and structure of CNTs. Higher temperatures generally promote the formation of high-quality nanotubes but may also lead to defects if not controlled properly.
- The choice of carbon source gas and the presence of additional gases (e.g., hydrogen or argon) influence the growth rate and morphology of the nanotubes.
-
Catalyst Particle Size and Shape:
- The size of the catalyst nanoparticles determines the diameter of the resulting nanotubes. Smaller particles produce narrower nanotubes.
- The shape and crystallographic orientation of the catalyst particles also affect the chirality and structure of the nanotubes.
-
Challenges in Growth Control:
- Achieving uniform growth of CNTs with desired properties (e.g., specific diameter, chirality, and length) remains a challenge.
- Defects such as kinks, bends, and impurities can form during growth, affecting the mechanical and electrical properties of the nanotubes.
-
Applications and Future Directions:
- Understanding the growth mechanisms enables the development of tailored CNTs for applications in electronics, composites, energy storage, and biomedical devices.
- Ongoing research focuses on improving growth techniques to produce CNTs with precise control over their properties, paving the way for advanced technologies.
By delving into these key points, we gain a comprehensive understanding of how carbon nanotubes grow and how their growth can be optimized for specific applications.
Summary Table:
Key Aspect | Description |
---|---|
Catalytic Decomposition | Carbon-containing gases decompose on metal catalysts, releasing carbon atoms. |
Carbon Diffusion & Assembly | Carbon atoms form hexagonal rings, creating the nanotube's graphene-like walls. |
Growth Models | Tip-growth (catalyst at tip) or base-growth (catalyst anchored to substrate). |
Temperature & Gas Role | Higher temperatures and gas composition influence quality and morphology. |
Catalyst Size & Shape | Determines nanotube diameter, chirality, and structure. |
Challenges | Uniform growth, defect control, and property optimization remain key hurdles. |
Applications | Electronics, composites, energy storage, and biomedical devices. |
Learn how to optimize carbon nanotube growth for your applications—contact our experts today!