Carburization is a process that enhances surface hardness, wear resistance, and fatigue strength in metals. However, if not properly controlled, it can lead to several damages. These damages can significantly impact the quality and performance of the treated metal parts.
6 Key Issues to Avoid
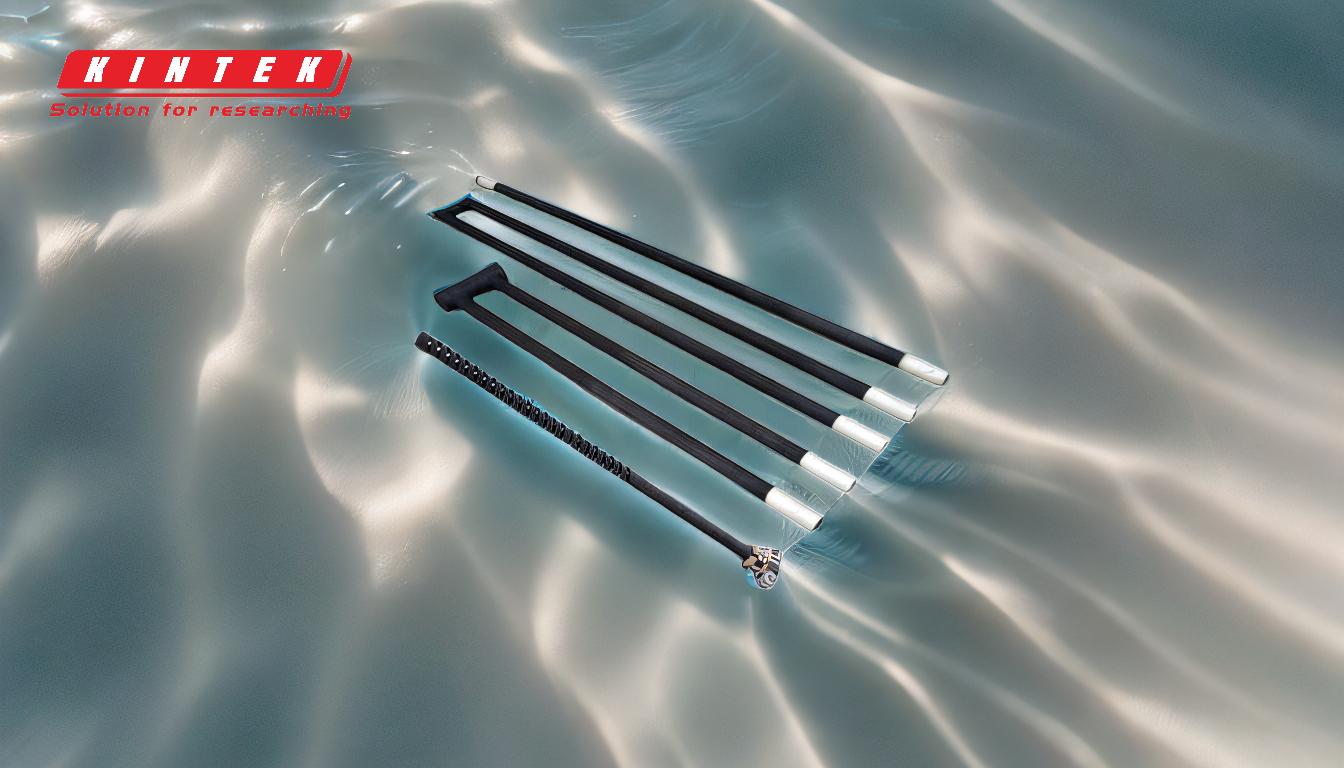
1. Retained Austenite
During carburization, the steel is heated into the austenite phase. If the cooling process is not controlled properly, some of the austenite may not transform into the desired martensite, leading to retained austenite. This can reduce the hardness and wear resistance of the surface, as austenite is softer than martensite.
2. Grain Boundary Oxidation
If the carbon potential is not controlled correctly, oxygen can penetrate the grain boundaries, leading to oxidation. This oxidation can weaken the grain boundaries and lead to premature failure under stress.
3. Intergranular Cracking
High carbon potential can also cause intergranular cracking. This occurs when the carbon concentration at the grain boundaries is too high, leading to localized embrittlement and cracking under stress.
4. Surface Cracking
Inadequate control of the carburizing process can result in surface cracking. This is often due to rapid cooling or uneven heating, which can cause stresses in the material that lead to cracks.
5. Low Surface Hardness
If the carbon potential is too low, the surface of the carburized part may not achieve the desired hardness. This can reduce the wear resistance and durability of the part.
6. Carbide Networking
An excessively high carbon potential can lead to the formation of carbides at the surface. These carbides can form a network that is brittle and can lead to premature failure under stress.
In addition to these direct damages, the process of atmosphere carburizing also has several disadvantages. These include the need for equipment conditioning after periods of inactivity, the reliance on empirical knowledge for repeatable results, and the requirement for large material allowances for post-processing operations. These factors contribute to variability in case depth and quality, and necessitate constant monitoring of environmental and safety issues.
Overall, while carburization is a valuable process for enhancing the properties of metals, careful control of the process parameters is essential to avoid these damaging effects and to ensure the desired properties are achieved.
Continue exploring, consult our experts
Discover how KINTEK SOLUTION's cutting-edge carburization solutions ensure the integrity and longevity of your metal parts. Our precision-engineered equipment and unparalleled process control minimize risks of retained austenite, grain boundary oxidation, intergranular cracking, surface cracking, and carbide networking. Ultimately, this leads to parts with superior surface hardness, wear resistance, and fatigue strength. Trust KINTEK SOLUTION for consistent results and unparalleled expertise in the carburization industry. Elevate your metal treatment game today!