Physical Vapor Deposition (PVD) is a sophisticated coating process used to deposit thin films of material onto a substrate. The process involves converting a solid target material into a vapor phase, which then condenses onto the substrate to form a thin, durable, and often highly specialized coating. PVD is widely used in industries such as electronics, optics, and manufacturing due to its ability to produce high-quality, corrosion-resistant, and temperature-tolerant coatings. The process typically includes four main steps: evaporation, transport, reaction, and deposition. Each step is carefully controlled to ensure the desired properties of the final coating, such as adhesion, thickness, and composition.
Key Points Explained:
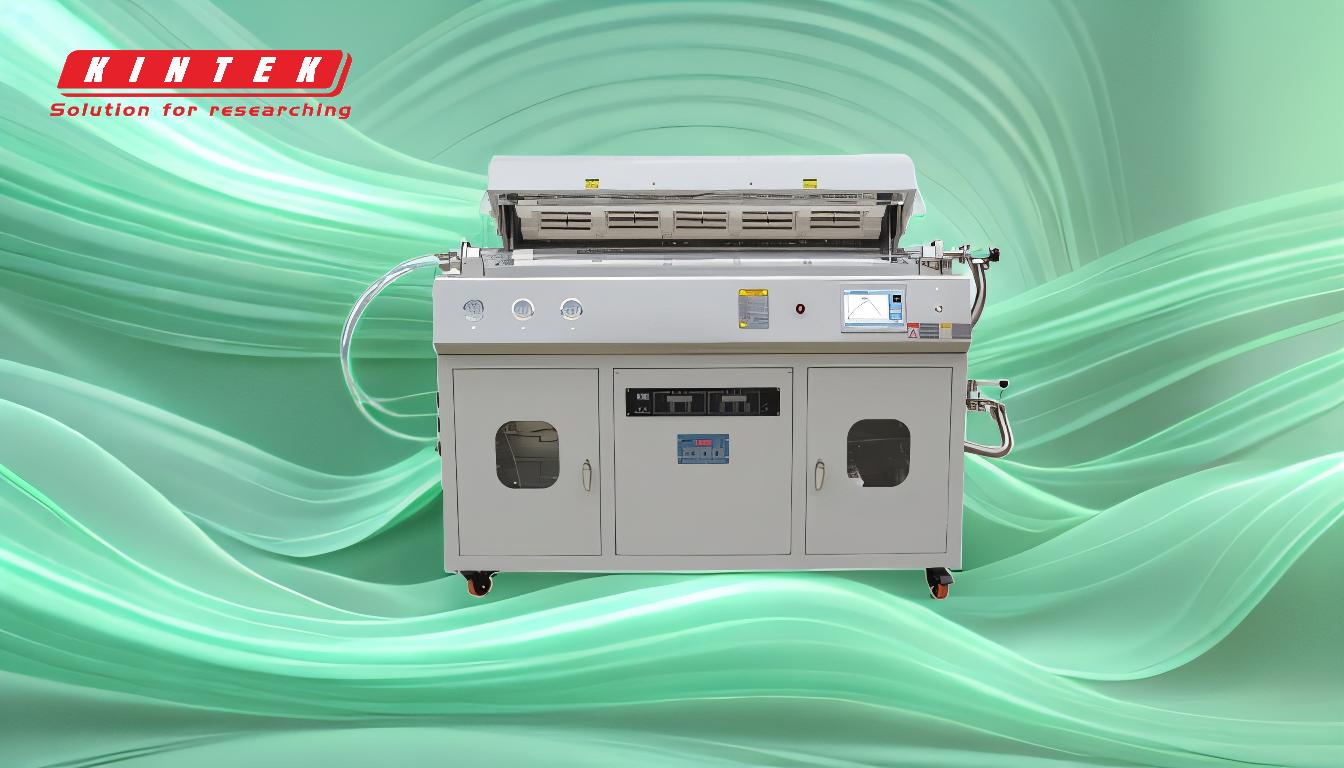
-
Evaporation of the Target Material:
- The first step in the PVD process is the evaporation of the target material. This is achieved by bombarding the solid target with a high-energy source, such as an electron beam, laser, or ion beam. The energy causes the atoms in the target to dislodge and transition from a solid to a vapor phase.
- The target material can be a metal, ceramic, or other solid substance, depending on the desired properties of the final coating. The evaporation process is typically carried out in a vacuum or low-pressure environment to minimize contamination and ensure a clean transfer of material.
-
Transport of Vaporized Atoms:
- Once the target material is vaporized, the atoms or molecules are transported through the reaction chamber towards the substrate. This transport occurs in a vacuum or low-pressure environment to prevent interference from background gases.
- The transport phase is critical because it determines how uniformly the vaporized material will reach the substrate. The "line-of-sight" method is often used, where the vaporized atoms travel directly from the target to the substrate without significant scattering.
-
Reaction (Optional):
- During the transport phase, the vaporized atoms may react with gases introduced into the chamber, such as oxygen or nitrogen. This reaction can form compounds like oxides, nitrides, or carbides, depending on the desired properties of the coating.
- For example, if a metal target is used and oxygen is introduced, the resulting coating may be a metal oxide. This step is optional and depends on the specific application and desired coating characteristics.
-
Deposition onto the Substrate:
- The final step is the deposition of the vaporized material onto the substrate. The atoms or molecules condense on the substrate surface, forming a thin film. The deposition process is controlled to ensure the desired thickness, adhesion, and uniformity of the coating.
- The substrate can be made of various materials, including metals, plastics, or ceramics, depending on the application. The deposition process often occurs at temperatures ranging from 50 to 600 degrees Celsius, depending on the materials involved and the desired properties of the coating.
-
Control and Monitoring:
- The PVD process is highly controlled to ensure the quality of the final coating. Parameters such as temperature, pressure, and deposition rate are carefully monitored and adjusted.
- Tools like quartz crystal rate monitors are used to measure and control the thickness of the deposited film. Additionally, the reaction chamber is often pumped down to very low pressures to minimize the presence of background gases that could interfere with the deposition process.
-
Advantages of PVD:
- Durability: PVD coatings are known for their hardness, wear resistance, and durability, making them suitable for high-stress applications.
- Corrosion Resistance: The coatings provide excellent protection against corrosion, even in harsh environments.
- High-Temperature Tolerance: PVD coatings can withstand high temperatures, making them ideal for applications in aerospace, automotive, and industrial settings.
- Versatility: PVD can be used to deposit a wide range of materials, including metals, ceramics, and composites, onto various substrates.
-
Applications of PVD:
- Electronics: PVD is used to deposit thin films in semiconductor devices, solar panels, and displays.
- Optics: The process is employed to create reflective or anti-reflective coatings on lenses and mirrors.
- Manufacturing: PVD coatings are applied to cutting tools, molds, and other components to enhance their performance and longevity.
In summary, the PVD process is a highly controlled and versatile method for depositing thin films with exceptional properties. By carefully managing each step—evaporation, transport, reaction, and deposition—manufacturers can produce coatings that meet the specific needs of a wide range of applications. The ability to control parameters such as thickness, adhesion, and composition makes PVD a valuable tool in modern manufacturing and technology.
Summary Table:
Step | Description |
---|---|
Evaporation | Target material is vaporized using high-energy sources like electron beams or lasers. |
Transport | Vaporized atoms travel through a vacuum or low-pressure environment to the substrate. |
Reaction | Optional step where vaporized atoms react with gases to form compounds like oxides. |
Deposition | Vaporized material condenses onto the substrate, forming a thin, durable coating. |
Control | Parameters like temperature, pressure, and deposition rate are carefully monitored. |
Advantages | Durability, corrosion resistance, high-temperature tolerance, and versatility. |
Applications | Electronics, optics, and manufacturing for enhanced performance and longevity. |
Discover how PVD can elevate your product performance—contact our experts today!