Hydrogen embrittlement is a phenomenon where metals, particularly high-strength steels, become brittle and fracture due to the absorption and diffusion of hydrogen atoms. Temperature plays a critical role in this process, influencing the solubility, diffusion rate, and trapping behavior of hydrogen in the metal lattice. At lower temperatures, hydrogen diffusion slows down, reducing the likelihood of embrittlement, but hydrogen atoms may accumulate at trap sites, increasing local stress concentrations. At higher temperatures, hydrogen diffusion accelerates, potentially leading to more uniform distribution but also increasing the risk of hydrogen-induced cracking under stress. Understanding the temperature-dependent behavior of hydrogen in metals is essential for mitigating embrittlement in industrial applications.
Key Points Explained:
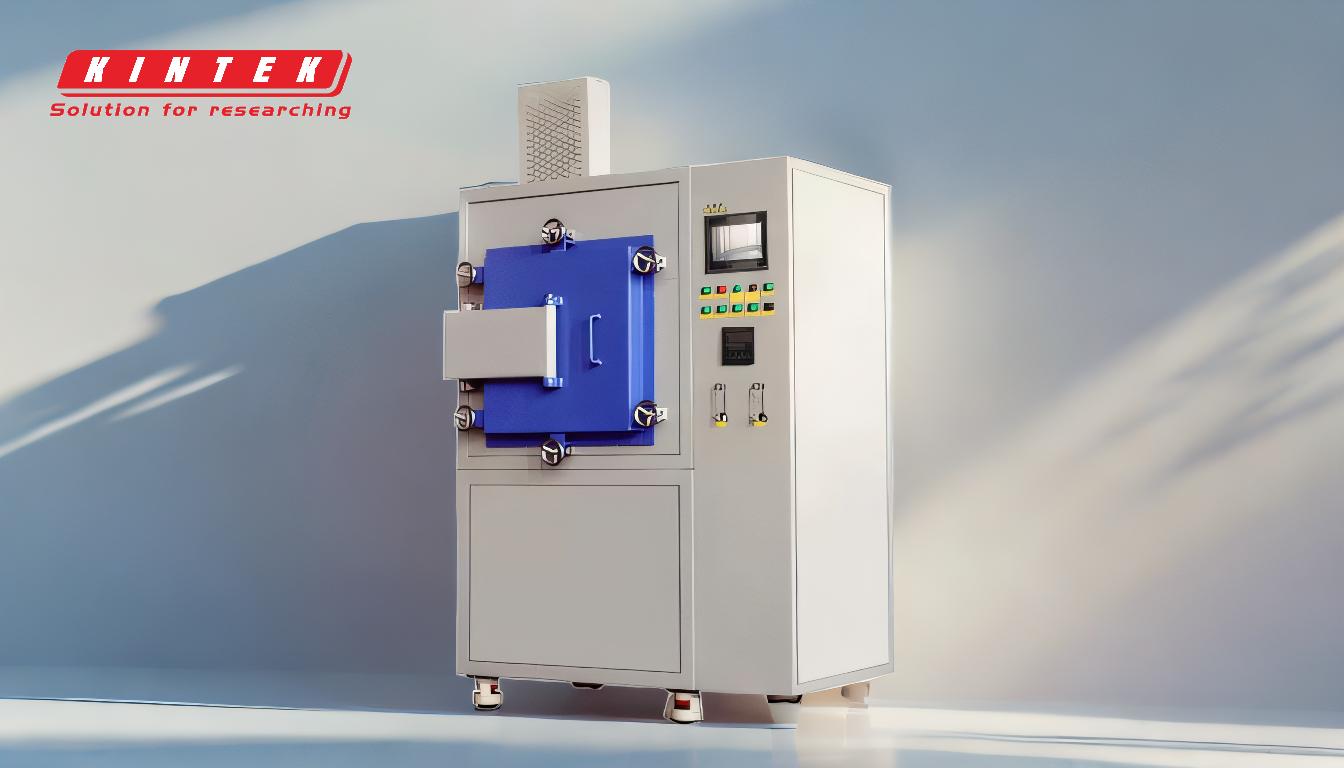
-
Hydrogen Solubility and Temperature:
- Hydrogen solubility in metals increases with temperature. At higher temperatures, more hydrogen can dissolve into the metal lattice, potentially increasing the risk of embrittlement.
- However, higher temperatures also enhance hydrogen diffusion, which can lead to a more uniform distribution of hydrogen, reducing localized stress concentrations that cause embrittlement.
-
Hydrogen Diffusion and Temperature:
- Hydrogen diffusion is thermally activated, meaning it increases exponentially with temperature. At elevated temperatures, hydrogen atoms move more freely through the metal lattice, which can either mitigate or exacerbate embrittlement depending on the stress conditions.
- At lower temperatures, hydrogen diffusion slows significantly, causing hydrogen atoms to accumulate at microstructural defects (e.g., grain boundaries, dislocations), which can lead to localized embrittlement.
-
Hydrogen Trapping and Temperature:
- Hydrogen atoms can be trapped at defects in the metal lattice, such as dislocations, vacancies, and grain boundaries. The strength of these traps is temperature-dependent.
- At lower temperatures, hydrogen atoms are more likely to remain trapped, increasing the risk of local embrittlement. At higher temperatures, hydrogen atoms can escape from these traps, potentially reducing local stress concentrations.
-
Temperature and Mechanical Properties:
- Temperature affects the mechanical properties of the metal itself, such as yield strength and ductility. Higher temperatures generally reduce yield strength and increase ductility, which can influence the susceptibility to hydrogen embrittlement.
- In some cases, higher temperatures can reduce the risk of embrittlement by allowing the metal to deform plastically rather than fracturing in a brittle manner.
-
Practical Implications for Material Selection and Design:
- For applications where hydrogen exposure is unavoidable, materials with lower hydrogen solubility and reduced sensitivity to trapping should be selected.
- Operating temperatures should be carefully controlled to balance hydrogen diffusion and solubility. For example, in high-temperature environments, materials with high resistance to hydrogen-induced cracking are preferred.
-
Experimental Observations and Case Studies:
- Studies have shown that hydrogen embrittlement is most severe at intermediate temperatures (e.g., room temperature to 200°C), where hydrogen diffusion is sufficient to reach critical concentrations but not high enough to distribute hydrogen uniformly.
- At very low temperatures (e.g., cryogenic conditions), hydrogen embrittlement is less pronounced due to reduced hydrogen mobility, but the metal itself may become more brittle due to reduced ductility.
-
Mitigation Strategies:
- Heat treatment processes, such as annealing, can be used to reduce residual stresses and modify the microstructure to minimize hydrogen trapping.
- Coatings and surface treatments can be applied to prevent hydrogen ingress into the metal.
- Environmental controls, such as reducing hydrogen partial pressure or using inhibitors, can also mitigate embrittlement.
By understanding the interplay between temperature and hydrogen embrittlement, engineers and material scientists can design more robust materials and processes to prevent catastrophic failures in hydrogen-exposed environments.
Summary Table:
Factor | Low Temperature | High Temperature |
---|---|---|
Hydrogen Solubility | Lower solubility, reduced risk of embrittlement | Higher solubility, increased risk of embrittlement |
Hydrogen Diffusion | Slower diffusion, hydrogen accumulates at trap sites | Faster diffusion, hydrogen distributes more uniformly |
Hydrogen Trapping | Stronger trapping, increased local stress concentrations | Weaker trapping, reduced local stress concentrations |
Mechanical Properties | Reduced ductility, higher risk of brittle fracture | Increased ductility, lower risk of brittle fracture |
Mitigation Strategies | Focus on reducing hydrogen ingress and optimizing microstructure | Use materials resistant to hydrogen-induced cracking and control operating conditions |
Need help mitigating hydrogen embrittlement in your materials? Contact our experts today for tailored solutions!