X-ray Fluorescence (XRF) is a powerful analytical technique used for elemental analysis, but it has limitations in detecting certain elements. The detection of elements by XRF depends on factors such as atomic number, fluorescence yield, and the energy of the emitted X-rays. While XRF can detect a wide range of elements, it struggles with light elements (low atomic number) due to their weak fluorescence signals and absorption issues. Additionally, elements with overlapping energy peaks or those present in trace amounts may also be challenging to detect accurately. Despite advancements like thinner beryllium windows and AI-driven calibration, XRF cannot reliably detect elements like hydrogen, helium, lithium, beryllium, and boron, among others.
Key Points Explained:
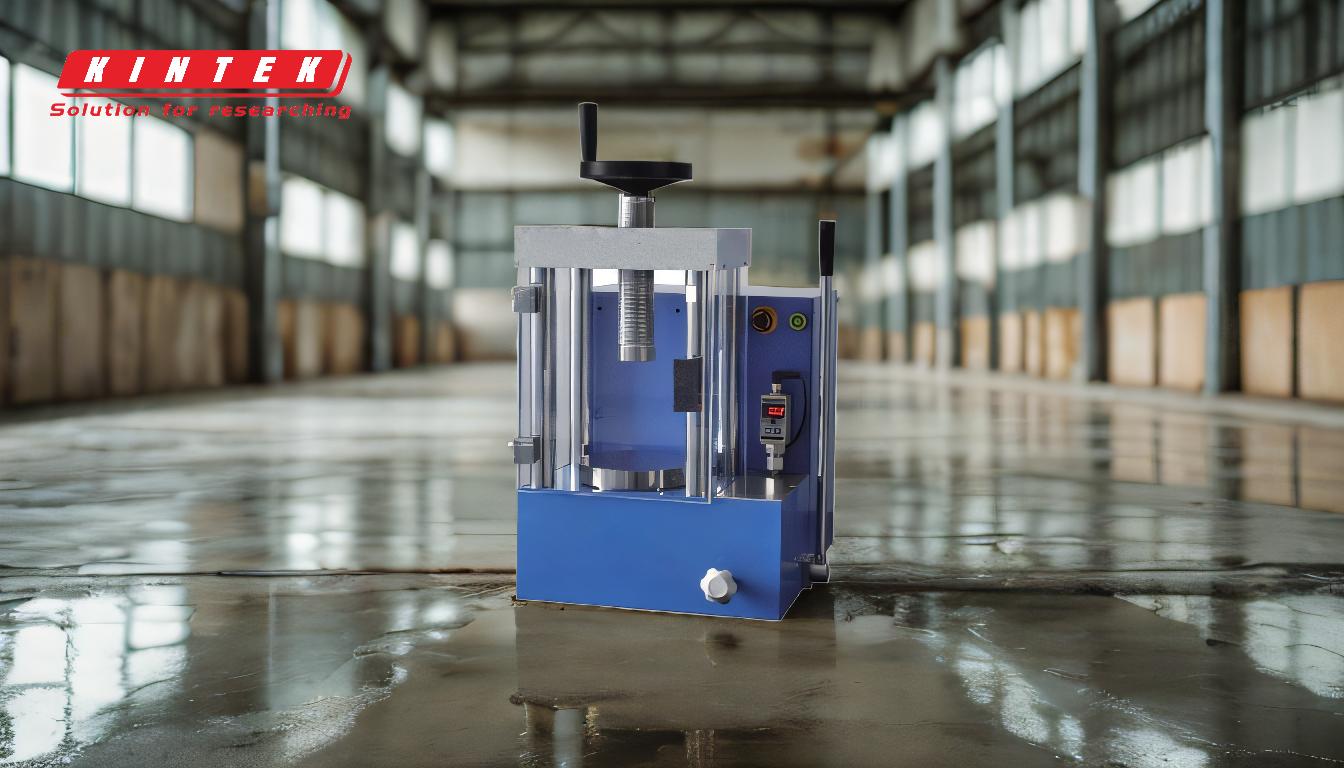
-
Detection Limits Based on Atomic Number:
- XRF is less effective for detecting light elements (low atomic number) due to their weak fluorescence signals. Elements like hydrogen (H), helium (He), lithium (Li), beryllium (Be), and boron (B) are particularly challenging because their X-ray emissions are either too weak or absorbed by air or the detector window.
- The beryllium window in XRF detectors, while necessary to protect the detector, also absorbs low-energy X-rays emitted by light elements, further limiting their detection.
-
Fluorescence Yield and Energy Overlap:
- The fluorescence yield (probability of X-ray emission) decreases with lower atomic numbers, making it harder to detect light elements.
- Elements with similar atomic numbers may have overlapping energy peaks, making it difficult to distinguish between them. For example, sulfur (S) and phosphorus (P) can sometimes interfere with each other’s detection.
-
Trace Element Detection:
- XRF is less sensitive to elements present in trace amounts (ppm or ppb levels). The detection limit varies depending on the element and the instrument's configuration, but trace elements like cadmium (Cd) or mercury (Hg) may not be detectable at very low concentrations.
-
Instrument Configuration and Advancements:
- While advancements like thinner beryllium windows, higher-power X-ray tubes, and AI-driven calibration improve detection limits, they cannot completely overcome the inherent limitations of XRF for certain elements.
- Ultra-coarse collimators and shorter distances between the X-ray tube and sample can enhance light element analysis but are not universally effective.
-
Non-Destructive Nature and Multi-Element Capability:
- Despite its limitations, XRF remains a valuable tool due to its non-destructive nature and ability to detect multiple elements simultaneously. This makes it ideal for applications like quality control, environmental monitoring, and material analysis.
-
AI and Machine Learning in XRF Analysis:
- AI and machine learning are being used to improve XRF analysis by optimizing calibration, reducing interference, and enhancing data interpretation. However, these technologies cannot fundamentally change the physics of X-ray fluorescence, meaning elements with inherently weak signals or overlapping energies will still pose challenges.
In summary, while XRF is a versatile and powerful analytical tool, it cannot reliably detect certain light elements, trace elements, or those with overlapping energy peaks. Understanding these limitations is crucial for selecting the appropriate analytical technique for specific applications.
Summary Table:
Category | Elements/Challenges |
---|---|
Light Elements | Hydrogen (H), Helium (He), Lithium (Li), Beryllium (Be), Boron (B) |
Trace Elements | Cadmium (Cd), Mercury (Hg) at very low concentrations |
Energy Overlap | Sulfur (S) and Phosphorus (P) interference |
Instrument Limitations | Absorption by beryllium windows, weak fluorescence yield for low atomic number elements |
Need help selecting the right analytical technique? Contact us today for expert advice!